2024-09-12
Shapeshifting micro-marvels Cornell
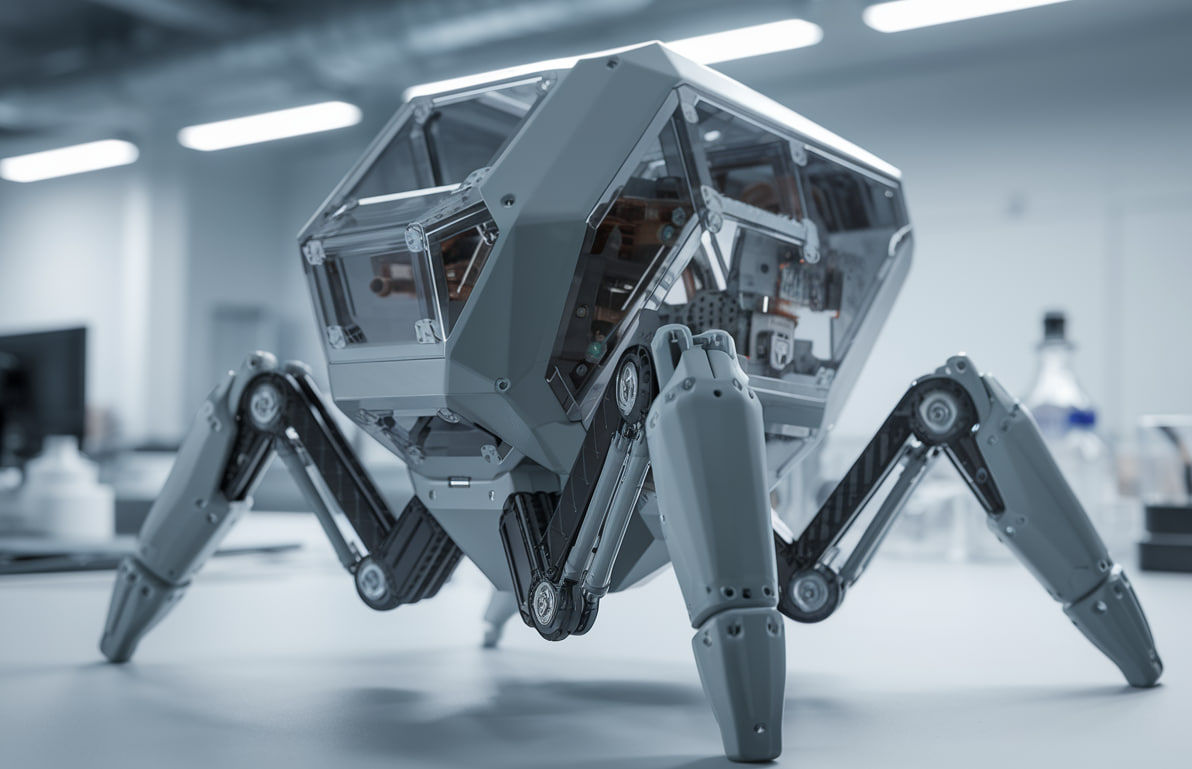
In a groundbreaking development that promises to revolutionize the field of microrobotics, researchers at Cornell University have unveiled a new class of microscale robots capable of transforming from flat sheets into complex 3D shapes and even crawling. This innovation, detailed in a recent paper published in Nature Materials, represents a significant leap forward in the creation of versatile, adaptable micro-machines.
The heart of this technological marvel lies in its unique design, which draws inspiration from kirigami, the Japanese art of paper cutting. Unlike its more famous cousin origami, which relies solely on folding, kirigami introduces strategic cuts to create intricate 3D structures from flat materials. The Cornell team, led by Professor Itai Cohen, has ingeniously applied this principle to the realm of microrobotics, resulting in what they call "metasheet robots."
These diminutive devices, measuring less than a millimeter in size, begin their existence as two-dimensional hexagonal sheets. However, their true potential is unlocked when subjected to electrical stimulation. With a carefully applied jolt of electricity, these metasheets transform into pre-programmed three-dimensional shapes, capable of not just maintaining these configurations but also locomoting across surfaces.
The key to this transformative ability lies in the metasheet's intricate structure. Each robot consists of approximately 100 silicon dioxide panels interconnected by over 200 actuating hinges. These hinges, astonishingly thin at just 10 nanometers, are the true workhorses of the system. When activated electrochemically, they create mountain and valley folds, splaying open and rotating the panels. This mechanism allows the robot to alter its coverage area and achieve local expansion and contraction of up to 40%.
What sets these metasheet robots apart is their unprecedented versatility. Depending on which hinges are activated, the robot can adopt a wide array of shapes, potentially even wrapping itself around other objects before unfolding back into its original flat configuration. This level of adaptability opens up a world of possibilities for microscale applications that were previously thought impossible.
The implications of this technology are far-reaching. As co-lead author Qingkun Liu points out, the inspiration came from living organisms that can change their shape – a capability that has long eluded traditional robotics. Most robots, once fabricated, are limited to moving certain limbs while maintaining a static overall shape. The metasheet robots shatter this limitation, offering a degree of flexibility and adaptability that more closely mimics biological systems.
Looking to the future, Professor Cohen and his team are already envisioning the next phase of metasheet technology. They aim to combine these flexible mechanical structures with electronic controllers, creating what they term "elastronic" materials. These materials would possess properties that transcend what's possible in nature, potentially revolutionizing fields ranging from micromachinery to biomedical devices.
One of the most exciting prospects is the development of materials that can respond to stimuli at nearly the speed of light, rather than the speed of sound. This could lead to ultra-responsive systems capable of reacting to impacts or changes in their environment with unprecedented speed and precision. Cohen paints a vivid picture of materials that could "run away" when prodded or push back with greater force than they experienced, fundamentally altering our understanding of how materials can interact with their environment.
The potential applications of this technology are vast and varied. In the realm of biomedical science, these metasheet robots could lead to the development of minimally invasive diagnostic and therapeutic tools capable of navigating the complex terrain of the human body. In manufacturing, they could enable the creation of self-assembling micro-components, revolutionizing the production of miniaturized devices.
Moreover, the ability of these robots to harvest energy from light opens up possibilities for self-powering systems, reducing the need for external power sources in microscale devices. This could be particularly beneficial in remote or hard-to-reach environments, where traditional power delivery methods are impractical.
As with any groundbreaking technology, the development of metasheet robots also raises intriguing questions about the future of materials science and engineering. By creating materials that can actively respond to and interact with their environment in programmed ways, the Cornell team is blurring the lines between passive materials and active machines. This could lead to a new paradigm in material design, where the distinction between structure and function becomes increasingly fluid.
The journey from concept to practical application is often long and fraught with challenges, but the potential of metasheet robots to transform multiple fields is undeniable. As researchers continue to refine and expand upon this technology, we may be witnessing the dawn of a new era in microscale engineering – one where the limitations of size and shape are no longer barriers to innovation, but gateways to unprecedented possibilities.
In conclusion, Cornell University's kirigami-inspired metasheet robots represent more than just a clever application of an ancient art form to modern technology. They embody a fundamental shift in how we conceive of and interact with materials at the microscale. As this technology continues to evolve, it promises to open up new frontiers in fields ranging from medicine to manufacturing, potentially reshaping our understanding of what's possible in the realm of the very small. The future of microrobotics, it seems, is not just small – it's flexible, adaptive, and full of transformative potential.
Share with friends:
Write and read comments can only authorized users
Last news